Stained
rat testis tissue by Rabbit anti-Insulin-like peptide-5,
C Peptide (H-035-47) |
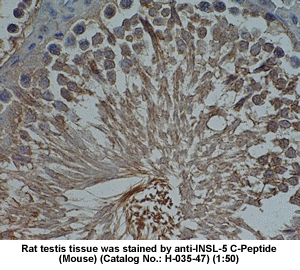
|
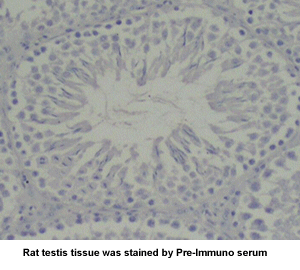
|
Stained
rat testis tissue by Rabbit anti-Insulin-like peptide-3,
C Peptide (H-035-48) |
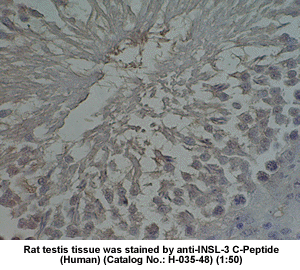
|
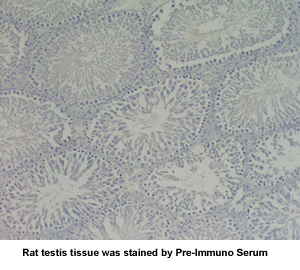
|
|
|
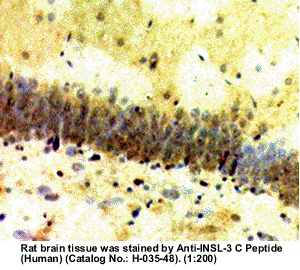
|
|
INSL
3, C Peptide Immunohistochemistry Protocol |
|
Slides
from Phoenix Biotech (Beijing) Co., LTD., |
Qsinghua
University, Beijing, P. R. China |
|
C-Peptide (Human) EIA Kit
(Catalog No.: EK-035-01) for Detection of C-Peptide
(Human) Levels in plasma or serum |
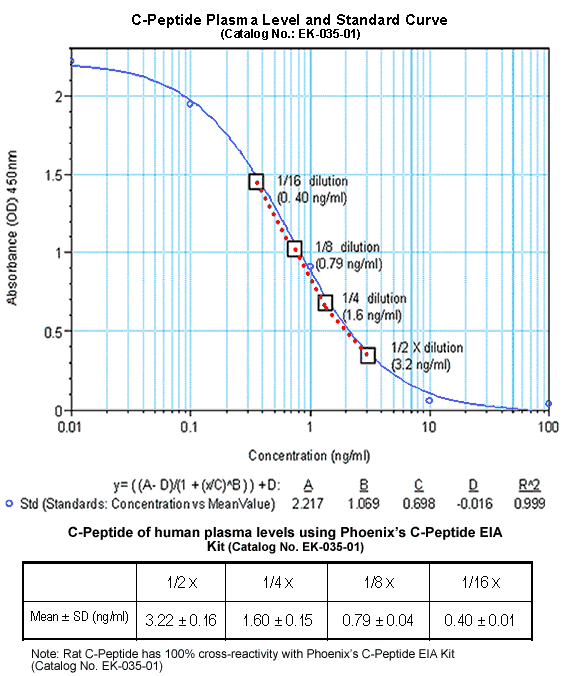
|
C-peptide exerts cardioprotective
effects in myocardial ischemia-reperfusion |
Ischemia followed by reperfusion
in the presence of polymorphonuclear leukocytes (PMNs)
results in cardiac dysfunction. C-peptide, a cleavage
product of proinsulin to insulin processing, induces
nitric oxide (NO)-mediated vasodilation. NO is reported
to attenuate cardiac dysfunction caused by PMNs after
ischemia-reperfusion (I/R). Therefore, we hypothesized
that C-peptide could attenuate PMN-induced cardiac dysfunction.
We examined the effects of C-peptide in isolated ischemic
(20 min) and reperfused (45 min) rat hearts perfused
with PMNs. C-peptide (70 nmol/kg iv) given 4 or 24 h
before I/R significantly improved coronary flow (P <
0.05), left ventricular developed pressure (LVDP) (P
< 0.01), and the maximal rate of development of LVDP
(+dP/dt(max)) compared with I/R hearts obtained from
rats given 0.9% NaCl (P < 0.01). N(G)-nitro-L-arginine
methyl ester (L-NAME) (50 micromol/l) blocked these
cardioprotective effects. In addition, C-peptide significantly
reduced cardiac PMN infiltration from 183 +/- 24 PMNs/mm(2)
in untreated hearts to 44 +/- 10 and 58 +/- 25 PMNs/mm(2)
in hearts from 4- and 24-h C-peptide-treated rats, respectively.
Rat PMN adherence to rat superior mesenteric artery
exposed to 2 U/ml thrombin was significantly reduced
in rats given C-peptide compared with rats given 0.9%
NaCl (P < 0.001). Moreover, C-peptide enhanced basal
NO release from rat aortic segments. These results provide
evidence that C-peptide can significantly attenuate
PMN-induced cardiac contractile dysfunction in the isolated
perfused rat heart subjected to I/R at least in part
via enhanced NO release. |
Young
LH, et al. Am J Physiol Heart Circ Physiol. 2000 Oct;279(4):H1453-9 |
|
Synergistic effects of C-peptide and insulin on coronary
flow in early diabetic rats |
The aims of the present study
are (1) to examine whether coronary flow is increased
and (2) to examine the role of C-peptide in relation
to nitric oxide (NO) production and coronary flow in
a rat heart (Wistar) during the early stages of type
1 diabetes. Coronary flow increased by 36.4% 10.6% (P
<.05) during the early stages of streptozotocin-induced
diabetes of isolated perfused rat hearts, but NO production
increased without significance. C-peptide alone did
not change coronary flow, but increased NO production
in diabetes. In the presence of insulin, C-peptide reversed
both flow and NO production to the control level of
normal rats (P <.05). In conclusion, during the early
stages of type 1 diabetes, coronary flow was increased,
and C-peptide in the presence of insulin synergistically
normalized the excessive flow and NO production induced
by C-peptide to the control level of normal rats. |
Nakamoto
H, et al. Metabolism. 2004 Mar;53(3):335-9 |
|
C-peptide and captopril are equally effective in lowering
glomerular hyperfiltration in diabetic rats |
BACKGROUND: C-peptide has
been shown to reduce glomerular hyperfiltration, glomerular
hypertrophy and urinary albumin excretion in type 1
diabetes, but its effect has not been compared with
that of an angiotensin-converting enzyme inhibitor (ACEI)
in the early stage of renal involvement in diabetes.
METHODS: Glomerular filtration rate (GFR) was measured
in terms of inulin clearance and renal blood flow, using
ultrasound technique, in four groups of streptozotocin-induced
diabetic rats before and after a 60 min infusion of
C-peptide (D-Cp), captopril (D-ACEI), C-peptide and
captopril (D-Cp-ACEI) or placebo (D-placebo). In addition,
a non-diabetic control group was studied before and
after captopril infusion (C-ACEI). RESULTS: GFR was
37-51% higher in the diabetic groups than in the control
animals. GFR decreased after treatment in the D-Cp,
D-ACEI and D-Cp-ACEI groups, but did not change in the
D-placebo group. Blood flow increased by 26-32% in the
three groups receiving captopril and by 5% in the diabetic
groups treated with C-peptide alone or placebo. The
increase in blood flow in the three ACEI-treated groups
was significantly greater than in the D-placebo group.
Filtration fraction fell significantly in all groups,
but only in the combined D-Cp-ACEI group did it fall
significantly more than in the D-placebo group. CONCLUSIONS:
C-peptide and captopril lower diabetes-induced glomerular
hyperfiltration to a similar extent, but the influence
of captopril on blood flow is greater than that of C-peptide,
suggesting different mechanisms of action. No statistically
significant additive effects of C-peptide and captopril
were shown in this acute infusion study. |
Samnegard
B, et al. Nephrol Dial Transplant. 2004 Mar 5 [Epub
ahead of print] |
|
Role of C-peptide in human physiology |
The C-peptide of proinsulin
is important for the biosynthesis of insulin but has
for a long time been considered to be biologically inert.
Data now indicate that C-peptide in the nanomolar concentration
range binds specifically to cell surfaces, probably
to a G protein-coupled surface receptor, with subsequent
activation of Ca(2+)-dependent intracellular signaling
pathways. The association rate constant, K(ass), for
C-peptide binding to endothelial cells, renal tubular
cells, and fibroblasts is approximately 3. 10(9) M(-1).
The binding is stereospecific, and no cross-reaction
is seen with insulin, proinsulin, insulin growth factors
I and II, or neuropeptide Y. C-peptide stimulates Na(+)-K(+)-ATPase
and endothelial nitric oxide synthase activities. Data
also indicate that C-peptide administration is accompanied
by augmented blood flow in skeletal muscle and skin,
diminished glomerular hyperfiltration, reduced urinary
albumin excretion, and improved nerve function, all
in patients with type 1 diabetes who lack C-peptide,
but not in healthy subjects. The possibility exists
that C-peptide replacement, together with insulin administration,
may prevent the development or retard the progression
of long-term complications in type 1 diabetes. |
Wahren
J, et al. Am J Physiol Endocrinol Metab. 2000 May;278(5):E759-68 |
|
Prevention of vascular and neural dysfunction in diabetic
rats by C-peptide |
C-peptide, a cleavage product
from the processing of proinsulin to insulin, has been
considered to possess little if any biological activity
other than its participation in insulin synthesis. Injection
of human C-peptide prevented or attenuated vascular
and neural (electrophysiological) dysfunction and impaired
Na+ and K+dependent adenosine triphosphate activity
in tissues of diabetic rats. Nonpolar amino acids in
the midportion of the peptide were required for these
biological effects. Synthetic reverse sequence (retro)
and all-D-amino acid (enantio) C-peptides were equipotent
to native C-peptide, which indicates that the effects
of C-peptide on diabetic vascular and neural dysfunction
were mediated by nonchiral interactions instead of stereospecific
receptors or binding sites. |
Ido
Y, et al. Science. 1997 Jul 25;277(5325):563-6 |
|
Specific binding of proinsulin
C-peptide to human cell membranes |
Recent reports have demonstrated
beneficial effects of proinsulin C-peptide in the diabetic
state, including improvements of kidney and nerve function.
To examine the background to these effects, C-peptide
binding to cell membranes has been studied by using
fluorescence correlation spectroscopy. Measurements
of ligand-membrane interactions at single-molecule detection
sensitivity in 0.2-fl confocal volume elements show
specific binding of fluorescently labeled C-peptide
to several human cell types. Full saturation of the
C-peptide binding to the cell surface is obtained at
low nanomolar concentrations. Scatchard analysis of
binding to renal tubular cells indicates the existence
of a high-affinity binding process with K(ass) >
3.3 x 10(9) M(-1). Addition of excess unlabeled C-peptide
is accompanied by competitive displacement, yielding
a dissociation rate constant of 4.5 x 10(-4) s(-1).
The C-terminal pentapeptide also displaces C-peptide
bound to cell membranes, indicating that the binding
occurs at this segment of the ligand. Nonnative D-C-peptide
and a randomly scrambled C-peptide do not compete for
binding with the labeled C-peptide, nor were crossreactions
observed with insulin, insulin-like growth factor (IGF)-I,
IGF-II, or proinsulin. Pretreatment of cells with pertussis
toxin, known to modify receptor-coupled G proteins,
abolishes the binding. It is concluded that C-peptide
binds to specific G protein-coupled receptors on human
cell membranes, thus providing a molecular basis for
its biological effects. |
Rigler R, et al. Proc Natl
Acad Sci U S A. 1999 Nov 9;96(23):13318-23. |
|
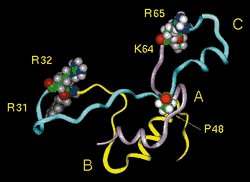
|
Human proinsulin
with its C-peptide. Insulin A chain (purple),
B chain (yellow), and C-peptide (blue). The CA and BC
junctions, the dibasic processing sites (R31-R32 and
K64-R65), are shown suitably poised for interaction
with the prohormone convertases . Pro48 (P48) corresponds
to Pro16 |
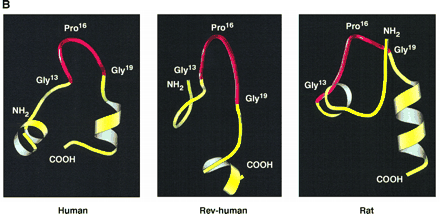
|
Supersecondary structures
of native human C-peptide (Human), reverse sequence
human C-peptide (Rev-human), and rat C-peptide 1 (Rat)
depicted by MolScript (16), based on predictions
by LINUS (14). A turn-like structure in the
midportion of the peptides (from C13 to C19)
is shown in red. Ido Y, et
al. Science. 1997 Jul 25;277(5325):563-6 |
|
|
|
|
Summary of effects of C-peptides
and fragments on 30 mM glucose-induced vascular
dysfunction (blood flow) in the skin chamber granulation
tissue model. All peptides were coadministered at
a concentration of 100 nM with 30 mM
glucose. Efficacy is expressed as an average
percent of the effect of 100 nM human C-peptide.
Because the reductions (by the peptides) in 30 mM
glucose-induced increases in 125I-albumin
permeation and blood flow were the same, only the blood
flow data are shown. The number in parentheses
to the right of each bar is the number of
chambers assessed. Scheffe's interval test
was used to assess differences (12). Significantly different
for 30 mM glucose: *P < 0.05.
Ido Y, et al. Science. 1997
Jul 25;277(5325):563-6 |
|
|
Ido
Y, et al. Science. 1997 Jul 25;277(5325):563-6
|
|
Linear representation of human proinsulin indicating
amino acid sequence of C-peptide and showing position
of COOH-terminal pentapeptide, which mimics action of
C-peptide in assays of binding and Na+-K+-ATPase
activity. Wahren J, et al.
Am J Physiol Endocrinol Metab. 2000 May;278(5):E759-68 |
|
Stimulation of Na+-K+-ATPase
activity (means ?nbsp;SE) in renal tubular segments
from rats by homologous C-peptide in different concentrations.
Wahren J, et al. Am J Physiol Endocrinol Metab. 2000
May;278(5):E759-68 |
Amino
acid sequences for different mammalian
C-peptides |
|
Human |
EAEDLQVGQVELGGGPGAGSLQPLALEGSLQ |
Monkey |
EAEDPQVGQVELGGGPGAGSLQPLALEGSLQ |
Bovine |
EVEGPQVGALELAGGPGAGG_____LEGPPQ |
Mouse-1 |
EVEDPQVEQLELGGSPG__DLQTLALEVARQ |
Rat-1 |
EVEDPQVPQLELGGGPEAGDLQTLALEVARQ |
Pig |
EAENPQAGAVELGGGLGG__LQALALEGPPQ |
Sheep |
EVEGPQVGALELAGGPG_____AGGLEGPPQ |
Horse |
EAEDPQVGEVELGGGPGLGGLQPLALAGPQQ |
|
Lines indicate gap
positions. |
|
|
Binding
of rhodamine-labeled C-Peptide (Human)
to human rental tubular cells |
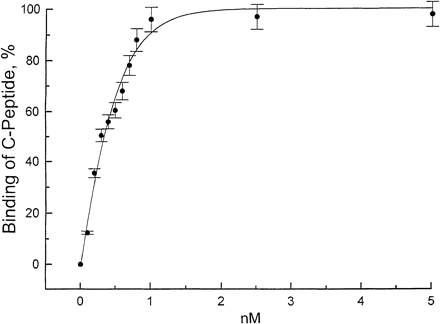
|
Binding of rhodamine-labeled
human C-peptide to human renal tubular cells as evaluated
by fluorescence correlation spectroscopy (FCS) in concentration
range of 0-5 nM. Association rate constant (Kass)
for C-peptide binding was 3.3 ?nbsp;109
M 1.
Values are means ?nbsp;SE shown as %. Wahren J,
et al. Am J Physiol Endocrinol Metab. 2000 May;278(5):E759-68 |
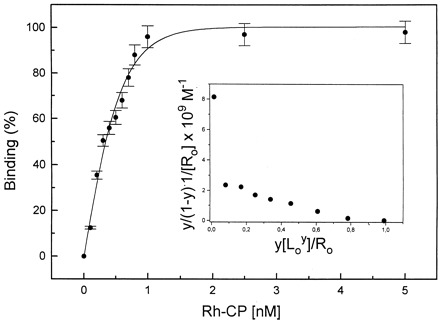
|
C-peptide
binding curve.
Binding of Rh-labeled C-peptide
to cell
membranes of renal tubular cells.
Fractional
saturation of the membrane-bound Rh-CP
(y) as a function
of the ligand concentration
(L) in the binding medium. Each
data point represents the mean of at least six measurements.
The binding curve
was simulated with Kass = 3.3 ?nbsp;109
M 1
and n = 1. Scatchard
plot is shown as Inset. Rigler R, et al. Proc
Natl Acad Sci U S A. 1999 Nov 9;96(23):13318-23. |
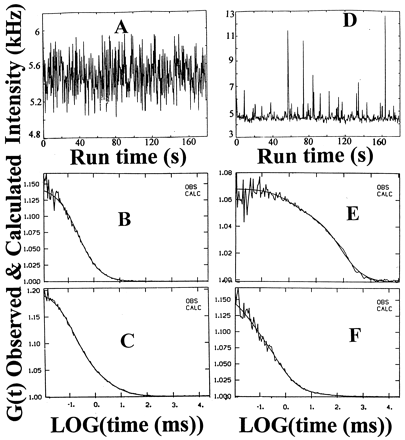
|
C-peptide binding and displacement
to the membranes of cultured renal tubular cells. Fluorescence
intensity fluctuations (A) and autocorrelation
function (B) for Rh-CP (5 nM) free in solution,
= 0.15 ms.
Fluorescence intensity fluctuations (D) and autocorrelation
function (E) for Rh-CP bound to membranes on
the cell surface. Diffusion times ( )
and corresponding fractions (y): 1 = 80 ms,
y1 = 0.75; 2 = 1 ms,
y2 = 0.15; 3 = 0.15 ms,
y3 = 0.1. Autocorrelation
functions of displacement of membrane bound Rh-CP by
postincubation of a thousandfold molar excess of nonlabeled
C-peptide (F) and nonlabeled C-terminal pentapeptide
(C). The observed and calculated data points
are completely overlapping (B, C, E,
and F). Rigler R, et al. Proc Natl Acad Sci U
S A. 1999 Nov 9;96(23):13318-23. |
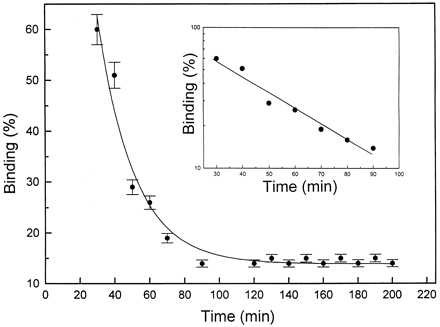
|
Time course of displacement
of Rh-CP by nonlabeled C-peptide. After incubation of
cells with 5 nM Rh-CP for 60 min, 5 µM
nonlabeled C-peptide was added, and FCS measurements
were carried out at given time intervals. Each data
point represents the mean of at least six measurements.
Log scale for the binding displacement process is shown
as Inset. Rigler R, et al. Proc Natl Acad Sci
U S A. 1999 Nov 9;96(23):13318-23. |
Table
Binding of C-peptide and insulin
to different cell types |
|
Ligand |
Cells |
Receptors/µm2* |
Kass,
109 M 1 |
|
C-peptide |
Renal tubular |
75 ?nbsp;12 |
3.3 |
|
Fibroblasts |
55 ?nbsp;10 |
2.5 |
|
Endothelial |
43 ?nbsp; 4 |
2.0 |
Insulin |
Renal tubular |
200 ?nbsp;10 |
1.2 |
|
*
Calculated from the number of Rh-C-peptide
or Rh-insulin binding sites in the
volume element with an area of 0.196 µm2. |
|
Rigler R, et al. Proc Natl
Acad Sci U S A. 1999 Nov 9;96(23):13318-23. |
|
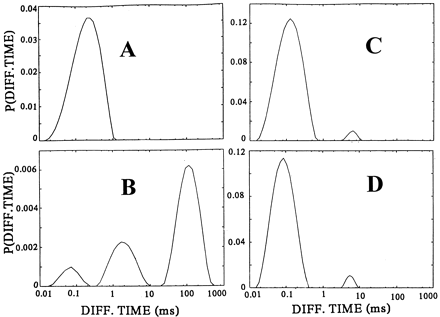
|
CONTIN
distributions of diffusion times P( i)
of C-peptide binding and displacement to the membranes
of cultured renal tubular cells. Rh-CP free in the incubation
medium (A), binding of Rh-CP to the cell membranes
(B), displacement of membrane-bound Rh-CP by
incubation with a thousandfold molar excess of nonlabeled
C-peptide (C), and inhibition of membrane binding
of Rh-CP after pretreatment of the cells with pertussis
toxin (D). Rigler R, et al. Proc Natl Acad Sci
U S A. 1999 Nov 9;96(23):13318-23. |
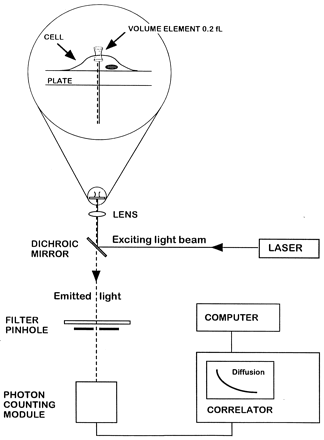
|
FCS experimental setup.
Light from an argon ion laser is focused by means of
a dichroic mirror and a lens to form a small volume
element (0.2 fl). The laser beam is projected from
below into a well containing a monolayer of cultured
cells and tetramethylrhodamine (Rh)-labeled ligand (see
magnified diagram at the top). After excitation of the
labeled ligand, emitted light is transmitted via the
dichroic mirror, a bandpass filter, and a pinhole to
a photodetector. The volume element is positioned onto
the cell surface with a microscope for detection of
ligand binding. The dimensions of the laser beam focus
and the pinhole together define the confocal volume
element. The detector signal is fed into a digital signal
correlator, which calculates the autocorrelation function
of the detected intensity fluctuations. Rigler R, et
al. Proc Natl Acad Sci U S A. 1999 Nov 9;96(23):13318-23. |
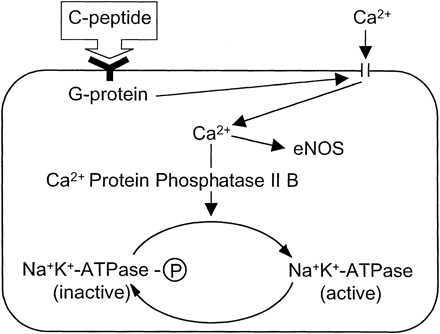
|
Available data are compatible
with the hypothesis that C-peptide
binds to cell membrane receptors coupled to a pertussis
toxin-sensitive G protein. The G protein activates Ca2+
channels, resulting in an increased intracellular Ca2+
concentration and activation of both endothelial nitric
oxide synthase (eNOS) and Ca2+-calmodulin-dependent
protein phosphatase 2B (PP2B). PP2B subsequently converts
the phosphorylated form of Na+-K+-ATPase
into its dephosphorylated, active form. Wahren
J, et al. Am J Physiol Endocrinol Metab. 2000 May;278(5):E759-68 |
|
|
Initial and final left ventricular
developed pressure (LVDP) expressed in mmHg in isolated
perfused rat hearts before ischemia and after reperfusion.
Hearts were perfused in the presence or absence of PMNs.
PMNs induced a significant contractile dysfunction,
which was attenuated by C-peptide. All values are expressed
as means ?nbsp;SE. Numbers of hearts are shown
at the bottom of the bars. **P < 0.01, ***P < 0.001 vs.
I/R + PMN + vehicle group.
Young LH, et al. Am J Physiol
Heart Circ Physiol. 2000 Oct;279(4):H1453-9 |
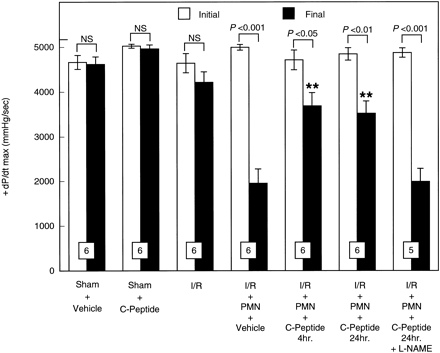
|
Initial and final maximal
rate of development of LVDP (+dP/dtmax)
expressed in mmHg/s in isolated perfused rat hearts
before ischemia and after reperfusion. Hearts were perfused
in the presence or absence of PMNs. PMNs induced a significant
contractile dysfunction, which was attenuated by C-peptide.
All values are expressed as means ?nbsp;SE. Numbers
of hearts are shown at the bottom of the bars. **P < 0.01 vs.
I/R + PMN + vehicle group.
Young LH, et al. Am J Physiol
Heart Circ Physiol. 2000 Oct;279(4):H1453-9 |
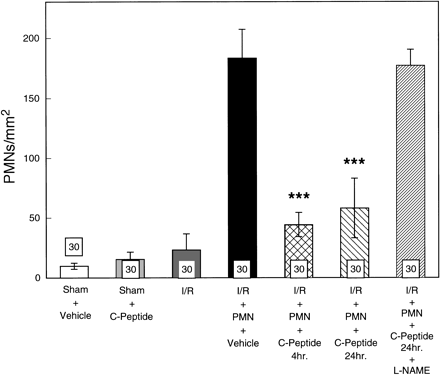
|
Histological assessment of
extravascular infiltrated PMNs in isolated perfused
rat heart samples taken from 3 rats per group and
10 areas per heart. All values are mean numbers
of PMNs/mm2 heart area ?nbsp;SE. The
number of PMNs infiltrated into postreperfusion cardiac
tissue was significantly attenuated by C-peptide. ***P < 0.001 vs.
I/R + PMN + vehicle group.
Young LH, et al. Am J Physiol
Heart Circ Physiol. 2000 Oct;279(4):H1453-9 |
|
Effect of C-peptide on basal
release of nitric oxide (NO) from isolated rat aortic
segments. C-peptide (70 nmol/kg) was injected intravenously
in rats 24 h before isolation of the aorta. All
values are means ?nbsp;SE of 12 segments isolated
from 4 rats/group. Young LH, et al. Am J Physiol
Heart Circ Physiol. 2000 Oct;279(4):H1453-9 |
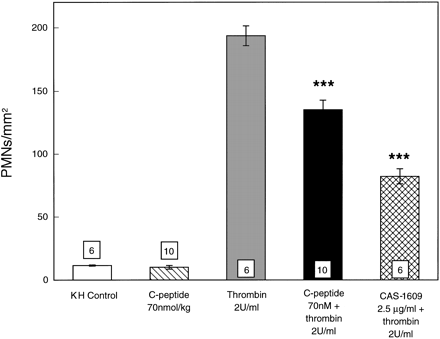
|
Adherence of rat PMNs
to rat superior mesenteric artery (SMA) endothelium
is expressed as PMNs/mm2. Rat SMAs were
isolated from rats given C-peptide or vehicle (0.9%
NaCl) and were either nonstimulated or stimulated
with thrombin (2 U/ml). PMNs that adhered to
endothelium were counted and analyzed. All values
are expressed as means ?nbsp;SE. Numbers shown
at the bottom of the bars indicate numbers of SMA
segments analyzed in each group. KH, Krebs-Henseleit
buffer. ***P < 0.001 vs.
thrombin group.
Young LH, et al. Am J Physiol
Heart Circ Physiol. 2000 Oct;279(4):H1453-9
|